Current Research Highlights
Schauer, Anna T. P., Regan, John, Glover, Simon C. O., Klessen, Ralf S.
The formation of direct collapse black holes under the influence of streaming velocitiesn MNRAS, 471, 4878 - 4884 (2017)
We study the influence of a high baryonic streaming velocity on the formation of direct collapse black holes (DCBHs) with the help of cosmological simulations carried out using the moving mesh code arepo. We show that a streaming velocity that is as large as three times the root-mean-squared value is effective at suppressing the formation of H2-cooled minihaloes, while still allowing larger atomic cooling haloes (ACHs) to form. We find that enough H2 forms in the centre of these ACHs to effectively cool the gas, demonstrating that a high streaming velocity by itself cannot produce the conditions required for DCBH formation. However, we argue that high streaming velocity regions do provide an ideal environment for the formation of DCBHs in close pairs of ACHs (the `synchronized halo' model). Due to the absence of star formation in minihaloes, the gas remains chemically pristine until the ACHs form. If two such haloes form with only a small separation in time and space, then the one forming stars earlier can provide enough ultraviolet radiation to suppress H2 cooling in the other, allowing it to collapse to form a DCBH. Baryonic streaming may therefore play a crucial role in the formation of the seeds of the highest redshift quasars.
Rahner, Daniel, Pellegrini, Eric W., Glover, Simon C. O., Klessen, Ralf S.
Winds and radiation in unison: a new semi-analytic feedback model for cloud dissolution MNRAS, 470, 4453 (2017)
Star clusters interact with the interstellar medium (ISM) in various ways, most importantly in the destruction of molecular star-forming clouds, resulting in inefficient star formation on galactic scales. On cloud scales, ionizing radiation creates H ii regions, while stellar winds and supernovae (SNe) drive the ISM into thin shells. These shells are accelerated by the combined effect of winds, radiation pressure, and SN explosions, and slowed down by gravity. Since radiative and mechanical feedback is highly interconnected, they must be taken into account in a self-consistent and combined manner, including the coupling of radiation and matter. We present a new semi-analytic 1D feedback model for isolated massive clouds (≥105 M⊙) to calculate shell dynamics and shell structure simultaneously. It allows us to scan a large range of physical parameters (gas density, star formation efficiency, and metallicity) and to estimate escape fractions of ionizing radiation fesc, i, the minimum star formation efficiency ɛmin required to drive an outflow, and recollapse time-scales for clouds that are not destroyed by feedback. Our results show that there is no simple answer to the question of what dominates cloud dynamics, and that each feedback process significantly influences the efficiency of the others. We find that variations in natal cloud density can very easily explain differences between dense-bound and diffuse-open star clusters. We also predict, as a consequence of feedback, a 4-6 Myr age difference for massive clusters with multiple generations.
Christian Baczynski, Simon C. O. Glover, Ralf S. Klessen
FERVENT: chemistry-coupled, ionizing and non-ionizing radiative feedback in hydrodynamical simulations MNRAS, 454, 380 (2015)
We introduce a radiative transfer code module for the magnetohydrodynamical adaptive mesh refinement code FLASH 4. It is coupled to an efficient chemical network which explicitly tracks the three hydrogen species H, H2, H+ as well as C+ and CO. The module is geared towards modelling all relevant thermal feedback processes of massive stars, and is able to follow the non-equilibrium time-dependent thermal and chemical state of the present-day interstellar medium as well as that of dense molecular clouds. We describe in detail the implementation of all relevant thermal stellar feedback mechanisms, i.e. photoelectric, photoionization and H2 dissociation heating as well as pumping of molecular hydrogen by UV photons. All included radiative feedback processes are extensively tested. We also compare our module to dedicated photodissociation region (PDR) codes and find good agreement in our modelled hydrogen species once our radiative transfer solution reaches equilibrium. In addition, we show that the implemented radiative feedback physics is insensitive to the spatial resolution of the code and show under which conditions it is possible to obtain well-converged evolution in time. Finally, we briefly explore the robustness of our scheme for treating combined ionizing and non-ionizing radiation.
Nicola Schneider, Volker Ossenkopf, Timea Csengeri, Ralf S. Klessen, Christoph Federrath, Pascal Tremblin, Philipp Girichidis, Sylvain Bontemps, Philippe André
Understanding star formation in molecular clouds. I. Effects of line-of-sight contamination on the column density structure A&A, 575, A79 (2015)
Mei Sasaki, Paul C. Clark, Volker Springel, Ralf S. Klessen, Simon C. O. Glover
Statistical properties of dark matter mini-haloes at z >= 15
Understanding the formation of the first objects in the Universe critically depends on knowing whether the properties of small dark matter structures at high redshift (z >= 15) are different from their more massive lower-redshift counterparts. To clarify this point, we performed a high-resolution N-body simulation of a cosmological volume 1 h-1 Mpc comoving on a side, reaching the highest mass resolution to date in this regime. We make precision measurements of various physical properties that characterize dark matter haloes (such as the virial ratio, spin parameter, shape, and formation times, etc.) for the high-redshift (z >= 15) dark matter mini-haloes we find in our simulation, and compare them to literature results and a moderate-resolution comparison run within a cube of side-length 100 h-1 Mpc. We find that dark matter haloes at high-redshift have a log-normal distribution of the dimensionless spin parameter centred around λ ∼ 0.03, similar to their more massive counterparts. They tend to have a small ratio of the length of the shortest axis to the longest axis (sphericity), and are highly prolate. In fact, haloes of given mass that formed recently are the least spherical, have the highest virial ratios, and have the highest spins. Interestingly, the formation times of our mini-haloes depend only very weakly on mass, in contrast to more massive objects. This is expected from the slope of the linear power spectrum of density perturbations at this scale, but despite this difference, dark matter structures at high-redshift share many properties with their much more massive counterparts observed at later times.
Erik Bertram, Rahul Shetty, Simon C. O. Glover, Ralf S. Klessen, Julia Roman-Duval, Christoph Federrath
Principal Component Analysis of Molecular Clouds: Can CO reveal the dynamics?
We use Principal Component Analysis (PCA) to study the gas dynamics in numerical simulations of typical MCs. Our simulations account for the non-isothermal nature of the gas and include a simplified treatment of the time-dependent gas chemistry. We model the CO line emission in a post-processing step using a 3D radiative transfer code. We consider mean number densities n_0 = 30, 100, 300 cm^{-3} that span the range of values typical for MCs in the solar neighbourhood and investigate the slope \alpha_{PCA} of the pseudo structure function computed by PCA for several components: the total density, H2 density, 12CO density, 12CO J = 1 -> 0 intensity and 13CO J = 1 -> 0 intensity. We estimate power-law indices \alpha_{PCA} for different chemical species that range from 0.5 to 0.9, in good agreement with observations, and demonstrate that optical depth effects can influence the PCA. We show that when the PCA succeeds, the combination of chemical inhomogeneity and radiative transfer effects can influence the observed PCA slopes by as much as ~ +/- 0.1. The method can fail if the CO distribution is very intermittent, e.g. in low-density clouds where CO is confined to small fragments.
Diane Cormier, Suzanne Madden, Vianney Lebouteiller, Frank Bigiel, and collaborators
The molecular gas reservoir of 6 low-metallicity galaxies from the Herschel Dwarf Galaxy Survey: A ground-based follow-up survey of CO(1-0), CO(2-1), and CO(3-2)
Observations of nearby starburst and spiral galaxies have revealed that molecular gas is the driver of star formation. However, CO, the most common tracer of this reservoir, is found faint in low metallicity star-forming galaxies, leaving us with a puzzle about how star formation proceeds in these environments. If the dearth of CO is probably due to photo-dissociation caused by a change in dust properties, radiation field and structure of the ISM, the actual molecular gas mass is not well known since low-metallicity galaxies can host a large amount of ``CO-dark'' molecular gas.
We have obtained CO(1-0), CO(2-1), and CO(3-2) observations in a subset of 6 galaxies from the Herschel Dwarf Galaxy Survey, with metallicities between 1/6 and 1/2 solar, to quantify their molecular gas reservoir and compare the molecular and atomic gas reservoirs to the star formation activity. We detect CO in 5 of the 6 galaxies and find that the CO luminosities are low while [CII], the typical PDR tracer, is bright in these galaxies. This results in [CII]/CO(1-0)~10 000, indicative of strong photodissociation effects.
The figure shows the Schmidt-Kennicutt relation for total gas, with spirals and starbursts from Kennicutt et al. (1998) and our dwarf galaxies, which are usually dominated by their HI gas. We measure their molecular gas masses from several methods: using the CO emission with a Galactic CO-to-H2 conversion factor XCO, gal, with a conversion factor dependent on metallicity XCO, Z (thus accounting for a CO-dark gas reservoir), and using the dust emission with a constant dust-to-gas mass ratio. The resulting masses are quite discrepant and stress the large uncertainties on the true molecular mass present in these compact galaxies. Given the unrealistically low mass values found with a Galactic XCO, those galaxies most likely host large amounts of CO-dark gas. Even with a metallicity-scaled XCO, the molecular depletion time scales are short (~0.7 Gyr), which would indicate, especially for Haro11, that they are efficient in forming stars and probably caught in a bursty episodic phase. We also constrain the physical conditions of the starburst galaxy Haro11 with IR-mm lines. By modeling its multiphase ISM with the spectral synthesis code Cloudy, we access its ISM mass distribution.
Dimitrios A. Gouliermis, Sacha Hony & Ralf S. Klessen (2014, MNRAS, 439, 3775)
The complex distribution of recently formed stars. Bimodal
stellar clustering in the star-forming region NGC 346
We present a detailed stellar clustering analysis with the
application of the two-point correlation function on distinct young
stellar ensembles. Our aim is to understand how stellar systems are
assembled at the earliest stages of their formation. Our object of
interest is the star-forming region NGC 346 in the Small Magellanic
Cloud. It is a young stellar system well revealed from its natal
environment, comprising complete samples of pre-main-sequence and
upper main-sequence stars, very close to their formation. The surface stellar density map constructed from the sample of young stars in the region of NGC 346 with the use of the Kernel Density Estimation technique is shown on the right. Isopleths at different density levels, drawn with different colours, signify the individual stellar clusterings identified in this region. The colour bar indicates different density levels in σ, the standard deviation of the measured stellar density in the whole region. North is up and east is to the left.
We apply a comprehensive characterization of the autocorrelation function for both centrally condensed stellar clusters and self-similar stellar distributions through numerical simulations of stellar ensembles. We interpret the observed autocorrelation function of NGC 346 on the basis of these simulations. This function, shown in the inset of the plot on the right, is well described by a broken power law, with the break occurring at a separation of 20.85 arcsec (~5.8 pc).
The autocorrelation function of NGC 346 can be best explained as the combination of two distinct stellar clustering designs, a centrally concentrated, dominant at the central part of the star-forming region and an extended self-similar distribution of stars across the complete observed field. The cluster component, similar to non-truncated young star clusters, is determined to have a core radius of ~2.5 pc and a density profile index of ~2.3. The extended fractal component is found with our simulations to have a fractal dimension of ~2.3, identical to that found for the interstellar medium, in agreement to hierarchy induced by turbulence.
This suggests that the stellar clustering at a time very near to birth behaves in a complex manner. It is the combined result of the star formation process regulated by turbulence and the early dynamical evolution induced by the gravitational potential of condensed stellar clusters.
J. Olofsson, L. Szűcs, Th. Henning, H. Linz, I. Pascucci, V. Joergens
The Herschel/PACS view of disks around low-mass stars in Chamaeleon-I
The understanding of the stellar mass dependence of protoplanetary disk parameters is necessary to complete our picture of disk formation and evolution. Furthermore, the stellar mass (in-)dependence of disk might result in very similar or very different planetary systems around low- and intermediate mass stars. Previous studies found longer disk lifetimes, lower accretion rates, and different organic chemistry in disks around low-mass stars and brown dwarfs, while other studies find stellar mass independent turbulent mixing strength, and similar disk scale heights.
In this study we aimed to complete the stellar mass spectrum between T Tauri stars and brown dwarfs by studying a number of young M dwarfs. We detected 17 members of the Chamaeleon-I star forming region in Herschel/PACS 70, 100 and 160 micron bands. The spectral energy distribution of each object was completed with archival data and a large grid of radiative transfer disk models were fitted to the observed SEDs. The main considered model parameters are the total dust mass, disk geometry and parameterized dust settling.
One important result from the modelling is that most of the detected disks -- in contrast with brown dwarf disks -- are not fully optically thin at far-IR wavelengths, thus only upper limit on the total dust mass could be determined. When combining our results with disk mass measurements from the literature (left figure: filled symbols correspond to masses derived from far-IR observations, while the rest is from sub-mm measurements), we find that disk mass estimates based on far-IR emission (including our work) tend to result in lower disk masses than those determined by sub-mm emission. In the case of more massive disks, this could be a consequence of optically thick emission and/or it could be because far-IR observations might miss cold dust in the outer disk.
We also find a correlation between the disk flaring and the mid- to far-IR colour index (see right figure). Higher flaring results in a flatter SED between the mid- and far-IR. The [11.56]-[100] = 0.5 colour index seems to separate flat and flared disks relatively well. Edge on disks (marked by green dimonds) might seem similar to flared disks or have much lower colour indexes (more flux at long wavelength).
Svitlana Zhukovska & Thomas Henning
Dust input from AGB stars in the Large Magellanic Cloud
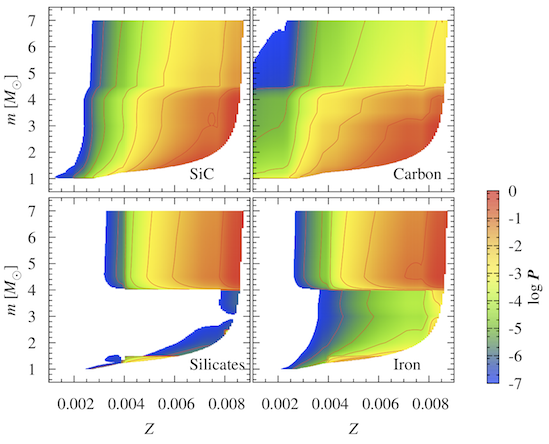
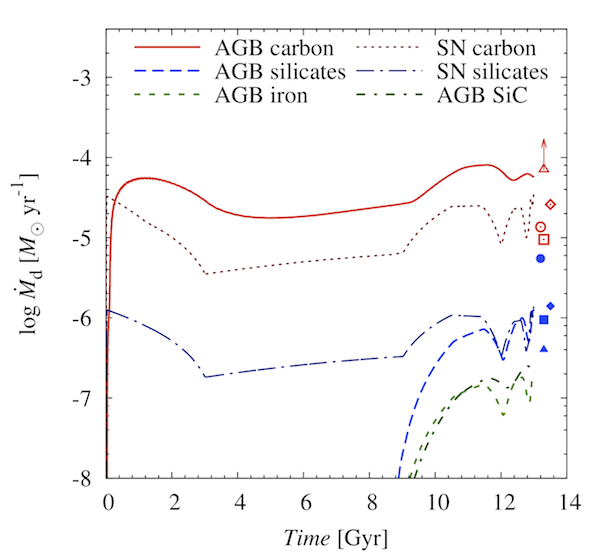
Tiny refractory solid particles or interstellar dust play an important role in physics of the interstellar medium (ISM) both in local galaxies and objects in the young universe. Circumstellar shells of the asymptotic giant branch (AGB) stars are well-known sites of efficient dust condensation, but their total contribution to the interstellar dust budget is still unclear. While AGB stars appear to play a minor role in the dust production in our Galaxy, they may be an important dust source in starburst galaxies in the young universe and in local objects with subsolar metallicities. The Large Magellanic Cloud (LMC) is an ideal local laboratory to investigate dust input from stars due to its proximity and position on sky.
We investigated the dust input from AGB stars during the LMC history with a model of dust evolution and mass- and metallicity-dependent dust yields. Time evolution of dust production rates by AGB stars in the LMC is shown in the left figure. It demonstrates the effect of galactic chemical evolution on the dust mixture from AGB stars. For the first 6 Gyr AGB stars return to the ISM an almost pure carbon dust mixture. Only when stars with higher metallicities enter the AGB stage of evolution, they eject silicate, iron and silicon carbide (SiC) grains to the ISM. For this reason, the initial metallicities of AGB stars that contributed to the current carbon dust population span over a much broader range than that of parent stars of other dust species shown in the right figure. It depicts the probability density that a star with initial metallicity Z and mass M contributed to the present grain population in the LMC. Moreover, the parent populations of silicate and metallic iron grains in the LMC consist mainly of intermediate mass stars (M>4Msun), while in the solar neighbourhood it has a bimodal distribution with a significant contribution from low mass stars. Such strong dependence of the dust formation in AGB stars on the initial metallicity results not only from a smaller amount of dust-forming atoms, but also from the different stellar evolution of low-mass stars at the AGB stage at low metallicity. We found that the contribution of AGB stars to the interstellar dust budget is higher in the LMC than in the Milky Way, but the total mass of grains from the AGB stars accumulated over the LMC history is significantly lower than the existing dust mass. Additional dust source such as growth by mantle accretion in the ISM on existing grains, which dominates in the Milky Way, is also required to reproduce the observed dust mass in the LMC.
"On the temperature structure of the Galactic Centre cloud G0.253+0.016"
Clark, Glover, Ragan, Shetty and Klessen, ApJL 2013
The Galactic Centre is a much harsher envrionment for star formation than is typical for the regions in the solar neighbourhood. Not only is the interstellar radiation field (ISRF) stronger in this region of the galaxy, but the region also has a higher cosmic ray flux - both of which are able to heat the gas and dust in the molecular clouds that form there. However, the true extent of these heat sources is unknown, with widely different values, obtained by a variety of methods, yielding quite different values for their strength.
However observations of the gas and dust can provide clues to the strength of the ISRF and cosmic ray flux. In particular, Galactic Centre cloud G0.253+0.016, more commonly referred to as The Brick (due to its exceptionally high density), has been observed to have very little star formation. Given its high density, the apparent lack of star formation activity is surprising. However it also means that the temperature of the cloud is more likely to be set by its environment, than any internal sources. In other words, we can use The Brick as thermometer, to probe the conditions in the Galactic Centre.
In this paper, we looked at how different levels of the ISRF and the cosmic-ray ionisation rate (CRIR) affect the gas and dust temperatures of a cloud that has similar properties to The Brick. The project uses a model of the ISM that accounts for the main heating and cooling processes, in addition to following some of the important chemistry (more details can be found in Glover and Clark 2012 and Clark et al. 2012).
We find that the ISRF must be around 1000 times the solar neighbourhood value, and the CRIR must be roughly 1E-14 /s, also roughly 1000 times the local value. For such high values of the CRIR, we find that cooling in the cloud's interior is dominated by neutral oxygen, in contrast to standard molecular clouds, which at the same densities are mainly cooled via CO. Our results suggest that the conditions near G0.253+0.016 are more extreme than those generally accepted for the inner 500 pc of the galaxy.
Rahul Shetty & Eve C. Ostriker
Maximally Star-forming Galactic Disks. II. Vertically Resolved Hydrodynamic Simulations of Starburst Regulation
We explore the self-regulation of star formation using a large suite of high-resolution hydrodynamic simulations, focusing on molecule-dominated regions (galactic centers and [U]LIRGS) where feedback from star formation drives highly supersonic turbulence. In equilibrium, the total midplane pressure, dominated by turbulence, must balance the vertical weight of the interstellar medium. Under self-regulation, the momentum flux injected by feedback evolves until it matches the vertical weight. We test this flux balance in simulations spanning a wide range of parameters, including surface density (Sigma), momentum injected per stellar mass formed (p*/m*), and angular velocity. The simulations are two-dimensional radial-vertical slices, and include both self-gravity and an external potential that helps to confine gas to the disk midplane. After the simulations reach a steady state in all relevant quantities, including the star formation rate Sigme_SFR, there is remarkably good agreement between the vertical weight, the turbulent pressure, and the momentum injection rate from supernovae. Gas velocity dispersions and disk thicknesses increase with p */m *. The efficiency of star formation per free-fall time at the midplane density, epsilon_ff(n_0), is insensitive to the local conditions and to the star formation prescription in very dense gas. We measure epsilon_ff(n_0) ~ 0.004-0.01, consistent with low and approximately constant efficiencies inferred from observations. For Sigma ~ (100-1000) M_sun pc^-2, we find Sigma_SFR in (0.1-4) M_sun kpc^-2 yr^-1, generally following a Sigma_SFR ~ Sigma^2 relationship. The measured relationships agree very well with vertical equilibrium and with turbulent energy replenishment by feedback within a vertical crossing time. These results, along with the observed Sigma-Sigma_SFR relation in high-density environments, provide strong evidence for the self-regulation of star formation.
Lukas Konstandin, Philipp Girichidis, Christoph Federrath, Ralf S. Klessen
A New Density Variance-Mach Number Relation for Subsonic and Supersonic Isothermal Turbulence
Understanding the intricate interplay between interstellar turbulence and self-gravity is one of the key problems in star formation theory. The supersonic turbulent velocity field is likely responsible for the complex and filamentary density structures observed in molecular clouds. It creates dense regions that can become gravitationally unstable and collapse into dense cores, and eventually turn into new stars. Statistical quantities describing this process, such as the initial mass function (IMF), the core mass function (CMF), and the star formation rate depend on the standard deviation of the density of the molecular cloud. The pioneering works of Padoan et al. (1997) and Passot & Vazquez-Semadeni (1998) have shown that the standard deviation of the probability density function of the mass density grows proportionally to the root-mean-square Mach number of the turbulent flow. We find that our measurements fit the linear relation between the rms Mach number and the standard deviation of the density distribution in a wide range of Mach numbers, where the proportionality constant depends on the type of forcing. In addition, we propose a new linear relation between the standard deviation of the density distribution and that of the velocity in compressible modes, i.e., the compressible component of the rms Mach number. In this relation the influence of the forcing is significantly reduced, suggesting a linear relation between the density and the compressible component of the rms Mach number, independent of the forcing, and ranging from the subsonic to the supersonic regime.
Jennifer Schober, Dominik R. G. Schleicher, Christoph Federrath, Simon Glover, Ralf S. Klessen, Robi Banerjee
Der Turbulente Dynamo und Nicht-ideale Magnetohydrodynamik in Primordialer Sternentstehung
Magnetfelder spielen eine wichtige Rolle im heutigen Universum. Sie erzeugen beispielsweise gigantische Jets bei Sternen und aktiven Galaxienkernen. Dennoch ist der Ursprung dieser starken Felder noch immer unklar, vor allem da im frühen Universum vermutlich nur schwache Magnetfelder während der Inflation und bestimmten Phasenübergängen oder durch Batterieprozesse erzeugt wurden.
Wir behaupten, dass der kleinskalige oder auch turbulente Dynamo diese Saatfelder auf dynamisch wichtige Werte verstärken kann, indem er turbulente kinetische Energie in magnetische Energie umwandelt. Dieser Prozess hängt stark von der Art der Turbulenz ab, die sich von inkompressibler Kolmogorov Turbulenz bis zu hoch kompressibler Burgers Turbulenz erstreckt. Turbulente Bewegungen strecken magnetische Flussröhren, was in der linken Abbildung gezeigt wird, und vergrössern dadurch die Magnetfeldstärke (siehe "stretch-twist-fold"-Mechanismus). Die Wachstumsrate der magnetischen Energie kann in bestimmten Grenzfällen aus der sogenannten Kazantsev-Theorie analytisch hergeleitet werden.
Der erste Zeitpunkt, zu dem ein Dynamo einsetzten konnte, ist während der Entstehung der ersten Sterne, da hier Turbulenz zum Beispiel durch Akkretion erzeugt wurde. Wir benutzen ein detailliertes chemisches Netzwerk und betrachten zusätzlich nicht-ideale magnetohydrodynamische Prozesse wie ambipolare Diffusion, um dem Kollapse des primordialen Gases zu folgen. Mit Hilfe der Wachstumsrate des turbulenten Dynamos können wir die Entwicklung der Magnetfeldstärke bestimmen.
Das Magnetfeld wächst am schnellsten auf der viskosen Skala, der kleinsten Skala des turbulenten Inertialbereichs. Wenn der Dynamo auf der viskosen Skala saturiert ist, wächst das Magnetfeld weiterhin auf größeren Skalen; das sogenannte nichtlineare Wachstum setzt ein. Die rechte Abbildung zeigt die Entwicklung der Magnetfeldstärke auf verschiedenen Längenskalen für die extremen Turbulenztypen. In beiden Fällen saturiert der Dynamo sehr schnell bezüglich der Teilchendichte und die turbulente Magnetfeldstärke erreicht Werte von der Größenordnung Mikro-Gauss.
Wir haben gezeigt, dass der turbulente Dynamo während dem Kollapse eines primordialen Halos dynamisch wichtige Magnetfelder erzeugen kann. Diese können sowohl die Entstehung als auch die Entwicklung der ersten Sterne und Galaxien bedeutend beeinflussen.
Rowan J. Smith, Rahul Shetty, Amelia M. Stutz, Ralf S. Klessen
Line Profiles of Cores within Clusters. I. The Anatomy of a Filament
Observations are revealing the ubiquity of filamentary structures in molecular clouds. As cores are often embedded in filaments, it is important to understand how line profiles from such systems differ from those of isolated cores. We perform radiative transfer calculations on a hydrodynamic simulation of a molecular cloud in order to model line emission from collapsing cores embedded in filaments. We model two optically thick lines, CS(2-1) and HCN(1-0), and one optically thin line, N2H+(1-0), from three embedded cores. In the hydrodynamic simulation, gas self-gravity, turbulence, and bulk flows create filamentary regions within which cores form. Though the filaments have large dispersions, the N2H+(1-0) lines indicate subsonic velocities within the cores.
We find that the observed optically thick line profiles of CS(2-1) and HCN(1-0) vary drastically with viewing angle. In over 50% of viewing angles, there is no sign of a blue asymmetry, an idealised signature of infall motions in an isolated spherical collapsing core. Profiles which primarily trace the cores, with little contribution from the surrounding filament, are characterised by a systematically higher HCN(1-0) peak intensity. The N2H+(1-0) lines do not follow this trend. We demonstrate that red asymmetric profiles are also feasible in the optically thick lines, due to emission from the filament or one-sided accretion flows onto the core. We conclude that embedded cores may frequently undergo collapse without showing a blue asymmetric profile, and that observational surveys including filamentary regions may underestimate the number of collapsing cores if based solely on profile shapes of optically thick lines.
Volker Gaibler, Sadegh Khochfar, Martin Krause, Joseph Silk
How do powerful AGN jets affect the star formation in massive galaxies?
Feedback from active galactic nuclei (AGN) has become a major component in simulations of galaxy evolution, in particular for massive galaxies. Since AGN jets have been shown to provide a large amount of energy and are capable to quench cooling flows, is is conjectured that they may cause stronger star formation at high redshift and reconcile at low redshift the simulated galaxy mass functions and galaxy colors with observations. The actual impact of radio jets on the host galaxy, however, is still not understood. Subgrid models of AGN activity in a galaxy evolution context so far have mostly focused and assumed the quenching of star formation.
To shed more light on the actual physics of the "radio mode" of AGN activity (feedback by jets), we have performed simulations of the interaction of a powerful AGN jet with the massive gaseous disc (1011 solar masses) of a high-redshift galaxy. We spatially resolve both the jet and the clumpy, multi-phase interstellar medium (ISM) and include an explicit star formation model in the simulation. Following the system over more than 107 years, we find that the jet activity excavates the central region, but overall causes a significant increase in star formation due to the formation of a blast wave with strong compression and cooling in the ISM. This impact is also evident in the shape of the density probability distribution function of the gas. The jet activity results in a ring- or disc-shaped population of young stars. At later times, the increase in star formation rate also occurs in the disc regions further out since the jet cocoon pressurizes the ISM of the galaxy. The total mass of the additionally formed stars may be up to 1010 solar masses for one duty cycle. We discuss the details of this jet-induced star formation and its potential consequences for galaxy evolution and observable signatures.
The left image is a tomographic view of the density, showing the galactic gas in blue/green and the jet in red/yellow 12 million years after the jet started and how the jet greatly affects the interstellar medium of the galaxy. The box size is 32 kpc. The right image shows a pseudo-color image of the stars formed in the simulation, also 12 million years after the jet started. Stars formed within the last million years are in blue; the older ones are either in red if they formed while the jet was still of, or in green if they formed while the jet was on. This shows the morphological signature of jet-induced star formation in the simulation. Supplementary movies of the simulations can be found at this website
Clark P.C., Glover S.C.O., Klessen R.S., Bonnell I.A., 2012, MNRAS
How long does it take to form a molecular cloud?
The life-cycle of molecular clouds sets the time-frame over which the star formation process can occur. As such, molecular cloud formation has received much attention in recent years, as we try to distinguish between theories for 'rapid' or `slow' star formation. In a simplified picture, the cycle can be thought of as comprising three distinct phases: assembly of the cloud, the formation of stars within the cloud, and the cloud's eventual dispersal (often linked to the termination of the star formation process). While we have made some progress on understanding the latter two stages of the cloud life-cycle the first stage is more uncertain, and has prompted much debate.
The idea of the colliding flow model for the formation of giant molecular clouds (GMCs) offers a simplified picture of how clouds can be assembled: two warm, marginally supersonic flows of gas collide head-on, and a dense molecular cloud builds up in the shocked layer. We have recently revisited this problem with a new treatment for the time-dependent chemistry that is coupled to a model of the ISM that accounts for the main heating and cooling processes.
We looked at two different colliding flows -- a slow flow with 6.8 km/s, and a fast flow with a velocity of 13.6 km/s. The clouds formed by the collision of these flows form stars, with star formation beginning after 16 Myr in the case of the slower flow, but after only 4.4 Myr in the case of the faster flow. In both flows, the formation of CO-dominated regions occurs only around 2 Myr before the onset of star formation. Prior to this, the clouds produce very little emission in the J (1-0) transition line of CO, and would probably not be identified as molecular clouds in observational surveys. In contrast, our models show that H2 dominated regions can form much earlier, with the timing depending on the details of the flow. In the case of the slow flow, small pockets of gas become fully molecular around 10 Myr before star formation begins, while in the fast flow, the first H2-dominated regions occur around 3 Myr before the first prestellar cores form. Our results are consistent with models of molecular cloud formation in which the clouds are dominated by 'dark' molecular gas for a considerable proportion of their assembly history.
The images in the figure show the evolution of the column number density (N), and the velocity-integrated intensity in the J (1-0) line of 12CO for the region in which the first star forms in each of the flows. Two times are shown: 2 Myr prior to star formation, and 0.8 Myr after the onset of star formation. The CO integrated intensity map is obtained via a radiative transfer calculation performed with the RADMC-3D code, and uses the large velocity gradient approximation to compute the CO level populations.
Girichidis, P., Federrath, C., Allison, R., Banerjee, R., Klessen, R.
Importance of the initial conditions for star formation - Statistical properties of embedded protostellar clusters
Stars in the present-day universe seem to form in a clustered environment rather than in isolation. Depending on the initial conditions of the system during the collapse of a star-forming region, the formation process of stars and stellar groups may differ significantly. Concentrated dense regions might even form only single stars or very compact stellar clusters. Initially flat regions are easily influenced by turbulent motions, leading to the formation of extended filaments. In this case, the formation of extended or even disconnected stellar clusters is much more likely than in concentrated density profiles. Depending on the formation mode as well as the spatial and temporal extend over which stars form, the initial dynamical state of the cluster is expected to differ. In addition, observations as well as numerical simulations show evidence mass segregation in young stellar clusters, i.e., high-mass and low-mass stars are not randomly distributed within the cluster. More massive stars are located closer to the centre of the cluster than their low-mass companions. Whether this mass segregation is primordial, i.e., the more massive stars form closer to the centre, or due to dynamical interactions, i.e., the segregation of masses via two-body relaxation, is still a matter of debate.
In this study we investigate the formation of stars and stellar clusters in dense molecular cloud cores with three-dimensional hydrodynamical simulations. We focus on the early dynamical evolution, the degree of substructure within the clusters as well as the interactions of the young stellar objects during the collapse of the cloud. In our simulations we vary the initial density profile as well as the initial turbulent velocities that are ubiquitous in star-forming regions.
In all setups the collapsing cloud virializes within the simulated time, which corresponds to a star formation efficiency of 20 per cent. Just considering the gas, all clouds have a virial or super-virial energy budget. Although the total mass of all protostars is only 20 per cent of the total cloud mass, their total kinetic energy is larger than that of the gas in the cases with multiple protostars. Analysing the entire stellar clusters as pure N-body systems, we find an overall sub-virial energy balance with the kinetic energy being roughly 20 per cent of the absolute total potential energy, independent of the varied initial conditions. If we concentrate on the central regions of the clusters (innermost 10-30 per cent of the protostars), we find virialized conditions (see picture on the right). The degree of subclustering strongly depends on the initial density profile. Initially uniform density allows for turbulent motions to form distinct subclusters before the global collapse can confine the gasinoneclusterinthecentralregion. The stronger the initial mass concentration around the centre of the cloud, the less subclustering is found. Bonnor-Ebert-like spheres show mainly one dominant central cluster with some substructure. The considered power-law density distributions form more compact protostellar clusters with less internal structure, if they form clusters at all. The degree of mass segregation varies strongly between the clusters; however, no cluster with significant inverse mass segregation is found. The mass segregation is consistent with the time for dynamical mass segregation, so all the clusters had enough time for dynamical relaxation of the most massive objects in the cluster. In the simulated collapsing cores, primordial mass segregation is not necessarily required to achieve a significant mass segregation at the end of the simulation.
Konstandin, L., Federrath, C., Klessen, R., Schmidt, W., JFM, accepted
Statistical properties of supersonic turbulence in the Lagrangian and Eulerian frameworks
Knowledge of the statistical characteristics of turbulence is a key prerequisite for understanding turbulent flows for virtually all scales. While common terrestrial flows are incompressible, astrophysical flows are highly supersonic and compressible. For example, the birth of stars in the interstellar medium is thought to be controlled by supersonic turbulence. As turbulence is by definition a process characterized by a chaotic and irregularly fluctuating velocity field, there is a scale-dependent spatial and temporal correlation of fluid quantities. The scale-dependent energy, density and velocity distributions, and the dynamical evolution thereof, are strongly influenced by the properties of the turbulence. While large improvements have been made in the understanding of incompressible turbulence in the last few years, there are still open questions in our understanding of compressible turbulence. The non-local, inter-scale processes of compressible turbulence arising, for example, in shock fronts change the Richardson-Kolmogorov picture of the energy cascade of incompressible turbulence, where scale-locality is crucial for the existence of universal statistics in the inertial range. Basic questions in compressible turbulence such as the existence of the inertial range, the associated scaling laws, and the influence of intermittency are still open. Guided by the idea of basic physical quantities such as momentum and kinetic energy, there is a trend to use mass-weighted velocity increments to describe turbulence in a compressible medium. Since Lagrangian tracer particles are designed to follow the turbulent flow and thus the mass flux in numerical simulations, they are a powerful tool for studying compressible turbulence, with an intrinsic mass-weighting.
Here, we also consider the statistics of turbulence in the integral range, where temporal correlations of the velocity field are exponentially damped, and the structure functions saturate over several integral time scales. A complete understanding of the statistical properties in this range is necessary as a solid foundation for further study of the complex and complicated behaviour of turbulence in the inertial range. In this paper we shed some light on this neglected topic. We compute Lagrangian statistics of the density and velocity fields of supersonic turbulence, using data from high-resolution, three-dimensional grid-simulations of driven turbulence with tracer particles. By calculating probability density functions and structure functions, we analyse the sensitivity of compressible turbulence on the forcing of the turbulence. In particular, we compare two limiting cases of purely solenoidal (divergence-free, shown in the picture) and purely compressive (curl-free) forcing. We present a detailed analysis of the scaling properties of the velocity structure functions and intermittency in the inertial subrange. We show that a simple analytic formula describes the saturated structure functions of all orders in the integral range, with the r.m.s. Mach number as single input parameter.
Allison, R. J., 2012, MNRAS, accepted
Testing the initial conditions and dynamical evolution of star clusters using Gaia - I
How stars form is one of the outstanding questions in astronomy. In particular, it is not clear if stars typically form in clusters, in particular in a quasi-static way, or form in a hierarchical distribution which can then dynamically evolve into dense star clusters. Dynamical interactions rapidly erase initial substructure and any memory of the initial conditions, and so deducing the initial conditions from dynamically evolved clusters and distinguishing these possibilities is difficult. Understanding the very early evolution of star clusters is also important as rapid dynamical evolution can have a profound influence on the clusters ability to erase substructure, dynamically mass segregate, process its binary star population and even form high multiplicity systems, such as the Trapezium in the Orion Nebula Cluster. Therefore it is important to be able to estimate whether a cluster is dynamically evolved or not; but this is difficult to measure as most potential indicators of dynamical evolution (e.g. mass segregation) can have a primordial origin. Escaping stars, however, offer a unique measure of the past dynamical state of a star cluster. Clusters with a violent past could be distinguishable from clusters with a relatively quiet history from their escaper population.
The ESA Gaia mission will conduct an all-sky astrometric and spectrophotometric survey of 10^9 point sources between 6th and 20th magnitude. The Gaia mission will be able to measure the distance, position and velocity distribution of the local Galactic stellar population to high accuracy. Therefore Gaia will not only find co-moving groups of stars (dispersing clusters), but it will be able to identify escaping stars from clusters and measure their velocities at large (angular and physical) distances from their birth cluster. We can then use the properties of these escaping stars to infer the dynamical state of the host cluster.
We have conducted N-body simulations to investigate if escaping stars leave a signal, measurable by Gaia, which we can use to infer there dynamical state.We present two different cluster initialisations: 1) an initially cool and highly structured cluster; and 2) an initially virialised and less structured cluster. The spatial distributions of the clusters after 4 Myrs of dynamical evolution are presented in the figures (cool and clumpy, top; Virialised and less clumpy, bottom).
We find that in the main body of the two clusters there is very little difference, but at larger distances form the cluster (i.e.the escaper population) the differences become very clear. We find that cool and clumpy clusters eject as many as 5 times as many stars, and that these ejected stars tend to have much higher velocities. These differences should be easily measurable by Gaia, therefore with Gaia the properties of escaping stars can be used to inform us of the initial conditions of star and cluster formation, and can provide us with a window into the dynamical history of star clusters.
Girichidis, P., Federrath, C., Banerjee, R., Klessen, R.
Importance of the initial conditions for star formation - Fragmentation-induced starvation and accretion shielding
The current paradigm of star formation suggests that most stars form in groups rather than in isolation. Whereas massive stars only seem to form in a dense environment, low-mass stars form in all observed star-forming regions. Numerical simulations suggest the formation of massive stars simultaneously with the formation of a cluster. The spatial distribution of stars in the forming cluster shows a dependence on the stellar mass: more massive stars are located closer to the centre of the group, low-mass stars tend to populate the outer regions. Whether this mass segregation is primordial and therefore determined by the formation scenario or due to a dynamical relaxation process is still a matter of debate and might differ significantly among different clusters Commonly found in observations as well as in numerical simulations is a universal distribution of masses initial mass function. Yet it is unclear, what is the influence of various physical processes and initial conditions on the initial mass distribution in star-forming regions. In particular the accretion processes within a cluster might significantly change the stellar mass distribution. As the formation process of a cluster in a dense environment is highly turbulent and dynamic, analytic estimates only give a vague idea of how accretion in these surroundings may work. With the help of simulations, two different cases have been proposed. In one scenario, which is called competitive accretion, the formation of the most massive stars is due to a privileged position close to the centre of the stellar cluster, where the accretion rates are predicted to be highest throughout the simulation. The other scenario is fragmentation-induced starvation, in which initially the central accretion rates are highest as well. However, subsequent fragmentation shows an increasing impact of the nascent protostars on the accretion behaviour of the central region that gets starved of material by the surrounding companions.
We investigate the impact of different initial conditions for the initial density profile and the initial turbulence on the formation process of protostellar clusters. We study the collapse of dense molecular cloud cores with three-dimensional adaptive mesh refinement simulations. We focus our discussion on the distribution of the gas among the protostellar objects in the turbulent dynamical cores. Despite the large variations in the initial configurations and the resulting gas and cluster morphology we find that all stellar clusters follow a very similar gas accretion behaviour. Once secondary protostars begin to form, the central region of a cluster is efficiently shielded from further accretion. Hence, objects located close to the centre are starved of material, as indicated by a strong decrease of the central accretion rate. This fragmentation-induced starvation occurs not only in rotationally supported discs and filaments, but also in more spherically symmetric clusters with complex chaotic motions.
The picture on the right shows the formation of a dense cluster from a fragmenting disc as well as the mass of the protostars as a function of time. In the simulation the first star forms very early and can accrete mass at a high rate, corresponding to the increase in mass in the lower plot up to a time of roughly 14 kyr. After that, subsequent fragmentation of the disc leads to the formation of multiple stars, indicated by the vertical dashed line. The upper part of the figure shows the fragmenting disc at 14.8 kyr, where 6 stars have formed. Quickly after the formation of further stars, the accretion onto the first central star decreases substantially, resulting a roughly constant mass of the central star for times larger than 15 kyr. The nascent stars that form at larger radii from the centre in dense filaments efficiently shield the accretion streams onto the central star.
Allison, R. J., Hubber, D. A., Smith, R., Goodwin, S. P.
A hybrid SPH/N-body method for star cluster simulations
The formation and dynamical evolution of young star clusters is an important, but complex problem. Stars form in turbulent molecular clouds, often in groups of tens to many thousands of stars. The early evolution of star clusters is therefore a complicated picture with young stars moving within, and interacting with, a dynamic gas potential. Thus, to understand the processes involved in the early stages of cluster formation requires the combination of self-gravitating hydrodynamics and detailed N-body dynamics.
In this paper we present a combined hybrid hydrodynamic (using SPH) / N-body code, which we have implemented into the SPH code SEREN (Hubber et al. 2011). In this scheme we integrate SPH particles with a 2nd order leapfrog, and the stars with a 4th order Hermite. By deriving the equations of motion from Lagrangian mechanics we obtain a formally conservative combined SPH/N-body scheme. This new approach is designed to bridge the gap between computationally expensive hydrodynamic simulations of star formation and gas free N-body simulations. Here we show a test of the code's ability to model complex star-gas systems. The figure shows the supersonic collision of two star-gas Plummer spheres. The Plummer spheres have been set-up to have 5000 gas particles and 200 star particles; and gas-dominated such that 90% of the mass of the system is in gas. The figure shows the collision at the initial state (top); just after the collision (middle); the end of the simulation (bottom), stars are shown by white dots, the colour table shows the column density of the gas in code units.
In this gas dominated, supersonic case, at the point of the collision the gas forms a shock front at x = 0, where the gas is compressed and heats up. Meanwhile, the collision of the Plummer spheres occurs on a timescale which is much shorter that the stellar crossing time, so the stars do not react to the collision. As the relative velocity of the two clusters is much greater than the individual velocity dispersions the effects of two-body encounters are negligible and the two clusters pass through each other almost unperturbed. However, as the star and gas components have de-coupled due to the collision the gas-free stellar systems are now unbound and expand as their velocity dispersion is too high to maintain their initial configuration. This test shows that the new hybrid scheme is able to combine hydrodynamical effects with high accuracy N-body dynamics, and during these tests works as expected. This scheme is an important step in our understanding of the highly complex early phase of cluster formation, and will allow us to better understand to connections between star clusters and their natal gaseous envelopes.
Glover, S. C. O., & Clark, P. C., 2011, MNRAS, accepted
Is molecular gas necessary for star formation?
Recent observations have shown that in most galaxies, there is a good correlation between the surface density of molecular gas and the surface density of the star formation rate. This has been widely been interpreted as evidence for a causal link between molecular gas and star formation — in other words, the assumption is that stars can only form within molecular gas. In this paper, we challenge this assumption. We have performed several simulations of a dense cloud of interstellar gas with properties (density, size, etc.) similar to those of a small molecular cloud, using a series of different models of the cloud chemistry, ranging from one in which the formation of molecules is not followed and the gas is assumed to remain atomic throughout, to one that tracks the formation of both H2 and CO. We find that the presence of molecules in the gas has little effect on the ability of the gas to form stars. In the figure on the right, we show how the total mass incorporated into stars, the total number of stars (represented here by artificial sink particles) and the mean stellar mass all evolve with time in our runs. Some differences between the runs are apparent, but these are all relatively minor, and the qualitative behaviour is the same regardless of whether we start with fully molecular gas, or keep the gas atomic throughout the simulation.
We can understand why the molecules have so little effect by looking in detail at the effectiveness of different heating and cooling processes within the cloud. We find that at low densities, the gas is able to cool via C+ fine-structure emission almost as efficiently as via CO rotational line emission, while at higher densities, the main cooling process involves the transfer of energy from gas to dust, meaning that the presence of molecules is again unimportant. Cooling by H2 is particularly inefficient, accounting for as little as 1 percent of the overall cooling in the cloud. Rather than the chemical makeup, we find that the most important factor controlling the rate of star formation is the ability of the gas to shield itself from the interstellar radiation field. As this is also a prerequisite for the survival of molecules within the gas, our results support a picture in which molecule formation and the formation of cold gas are both correlated with the column density of the cloud — and thus its ability to shield itself — rather than being directly correlated with each other.
Shetty, R., Glover, S., Dullemond, C., Ostriker, E., Harris, A., Klessen, R., 2011, MNRAS, 415, 3253
Modelling CO emission - II. The physical characteristics that determine the X factor in Galactic molecular clouds
We investigate how the X factor, the ratio of the molecular hydrogen column density (NH2) to velocity-integrated CO intensity (W), is determined by the physical properties of gas in model molecular clouds (MCs). The synthetic MCs are results of magnetohydrodynamic simulations, including a treatment of chemistry. We perform radiative transfer calculations to determine the emergent CO intensity, using the large velocity gradient approximation for estimating the CO population levels. In order to understand why observations generally find cloud-averaged values of X = XGal ~ 2x10^20 cm-2 K-1 km-1 s, we focus on a model representing a typical Milky Way MC. Using globally integrated NH2 and W reproduces the limited range in X found in observations and a mean value X = XGal= 2.2x 10^20 cm-2 K-1 km-1 s. However, we show that when considering limited velocity intervals, X can take on a much larger range of values due to CO line saturation. Thus, the X factor strongly depends on both the range in gas velocities and the volume densities. The temperature variations within individual MCs do not strongly affect X, as dense gas contributes most to setting the X factor. For fixed velocity and density structure, gas with higher temperatures T has higher W, yielding X ~ T-1/2 for T =20-100 K. We demonstrate that the linewidth-size scaling relationship does not influence the X factor - only the range in velocities is important. Clouds with larger linewidths, regardless of the linewidth-size relationship, have a higher W, corresponding to a lower value of X, scaling roughly as X~ linewidth-1/2. The 'mist' model, often invoked to explain a constant XGal consisting of optically thick cloudlets with well-separated velocities, does not accurately reflect the conditions in a turbulent MC. We propose that the observed cloud-averaged values of X ~ XGal are simply a result of the limited range in NH2, temperatures and velocities found in Galactic MCs - a nearly constant value of X therefore does not require any linewidth-size relationship, or that MCs are virialized objects. Since gas properties likely differ (albeit even slightly) from cloud to cloud, masses derived through a standard value of the X factor should only be considered as a rough first estimate. For temperatures T ~ 10-20 K, velocity dispersions ~ 1-6 km s-1 and NH2 ~ 2-20x10^21 cm-2, we find cloud-averaged values X ~ 2-4 ~ 10^20 cm-2 K-1 km-1 s for solar-metallicity models.
Federrath, C., Sur, S., Schleicher, D. R. G., Banerjee, R., Klessen, R. S., The Astrophysical Journal, Volume 731, 62 (2011).
A New Jeans Resolution Criterion for (M)HD Simulations of Self-gravitating Gas: Application to Magnetic Field Amplification by Gravity-driven Turbulence
Cosmic structure formation is characterized by the complex interplay between gravity, turbulence, and magnetic fields. The processes by which gravitational energy is converted into turbulent and magnetic energies, however, remain poorly understood.
Here, we show with high-resolution, adaptive-mesh simulations that MHD turbulence is efficiently driven by extracting energy from the gravitational potential during the collapse of a dense gas cloud. Compressible motions generated during the contraction are converted into solenoidal, turbulent motions, leading to a natural energy ratio, E_sol/E_tot of approximately 2/3. We find that the energy injection scale of gravity-driven turbulence is close to the local Jeans scale. If small seeds of the magnetic field are present, they are amplified exponentially fast via the small-scale dynamo process. The magnetic field grows most efficiently on the smallest scales, for which the stretching, twisting, and folding of field lines, and the turbulent vortices are sufficiently resolved. We find that this scale corresponds to about 30 grid cells in the simulations. We thus suggest a new minimum resolution criterion of 30 cells per Jeans length in (magneto)hydrodynamical simulations of self-gravitating gas, in order to resolve turbulence on the Jeans scale, and to capture minimum dynamo amplification of the magnetic field. Due to numerical diffusion, however, any existing simulation today can at best provide lower limits on the physical growth rates.
We conclude that a small, initial magnetic field can grow to dynamically important strength on time scales significantly shorter than the free-fall time of the cloud.
Short summary of the paper by Joshua Suresh
Dopcke, Gustavo; Glover, Simon C. O.; Clark, Paul C.; Klessen, Ralf S.
The Effect of Dust Cooling on Low-metallicity Star-forming Clouds
The theory for the formation of the first population of stars (Pop III) predicts a IMF composed predominantly of high-mass stars, in contrast to the present-day IMF, which tends to yield stars with masses less than 1 M_Solar. The leading theory for the transition in the characteristic stellar mass predicts that the cause is the extra cooling provided by increasing metallicity and in particular the cooling provided at high densities by dust. The aim of this work is to test whether dust cooling can lead to fragmentation and be responsible for this transition. To investigate this, we make use of high-resolution hydrodynamic simulations.
We follow the thermodynamic evolution of the gas by solving the full thermal energy equation, and also track the evolution of the dust temperature and the chemical evolution of the gas. We model clouds with different metallicities, and determine the properties of the cloud at the point at which it undergoes gravitational fragmentation. We follow the further collapse to scales of an AU when we replace very dense, gravitationally bound, and collapsing regions by a simple and nongaseous object, a sink particle.
Our results suggest that for metallicities as small as 10^{-5} Z_Solar, dust cooling produces low-mass fragments and hence can potentially enable the formation of low mass stars.
We conclude that dust cooling affects the fragmentation of low-metallicity gas clouds and plays an important role in shaping the stellar IMF even at these very low metallicities.
We find that the characteristic fragment mass increases with decreasing metallicity, but find no evidence for a sudden transition in the behaviour of the IMF within the range of metallicites examined in our present study.
The figure shows number density map of a slice in the densest clump, and the sink formation time evolution, for the 40 million particles simulation, and Z = 10^{-4} Z_Solar. The box is 100AU x 100AU and the time is measured from the formation of the first sink particle.
Clark, P. C., Glover, S. C. O., Smith, R. J., Greif, T. H., Klessen, R. S. & Bromm, V., 2011, Science, 331, 1040
The Formation and Fragmentation of Disks around Primordial Protostars
The first stars in the universe were not as solitary as previously thought. In fact, they could have formed alongside numerous companions when the gas disks that surrounded them broke up during formation, giving birth to sibling stars in the fragments. These are the findings of studies performed with the aid of computer simulations by researchers at Heidelberg University’s Centre for Astronomy together with colleagues at the Max Planck Institute for Astrophysics in Garching and the University of Texas at Austin (USA). The group’s findings, being published in Science magazine, cast an entirely new light on the formation of the first stars after the Big Bang.
Stars evolve from cosmic gas clouds in a fierce and complex battle between gravity and internal gas pressure. The density of the gas increases due to its own gravitational pull. This causes the gas to heat up, as a consequence the pressure rises, and the compression process comes to a halt. If the gas manages to get rid of the thermal energy, compression can continue and a new star is born. This cooling process works especially well if the gas contains chemical elements like carbon or oxygen. Stars forming in this way are normally low in mass, like our Sun. But in the early universe these elements had yet to emerge, so the primordial cosmic gas could not cool down very well. Accordingly, most theoretical models predict the masses of primordial stars to be about a hundred times greater than that of the Sun.
Heidelberg astrophysicist Dr. Paul Clark and his colleagues investigated these processes with the help of very high resolution computer simulations. Their findings indicate that this simple picture needs to be revised and that the early universe was not only populated by huge, solitary stars. The reason is the underlying physics of the so called accretion disks accompanying the birth of the very first stars. The gas from which a new star forms rotates, and so the gas is unable to fall directly onto the star, but first builds up a disk-like structure. Only as a result of internal friction can the gas continue to flow onto the star. If more mass falls onto this disk than it can transport inwards, it becomes unstable and breaks into several fragments. So instead of forming just one star at the centre, a group of several stars is formed. The distances between some of the stars can be as small as that between the Earth and the Sun.
According to Dr. Clark, this realisation opens up exciting new avenues for detecting the first stars in the universe. In the final stages of their lives, binaries or multiple stellar systems can produce intense bursts of X-rays or gamma rays. Future space missions are being planned specifically to investigate such bursts from the early universe. It is also conceivable that some of the first stars may have been catapulted out of their birth group through collisions with their neighbours before they were able to accumulate a great deal of mass. Unlike short-lived high-mass stars, low-mass stars may survive for billions of years. Intriguingly, says Dr. Clark, `some low-mass primordial stars may even have survived to the present day, allowing us to probe the earliest stages of star and galaxy formation right in our own cosmic backyard.'
Together with Dr. Simon Glover and Dr. Rowan Smith, Dr. Paul Clark is a member of the star-formation research group headed by Prof. Dr. Ralf Klessen at Heidelberg University’s Centre for Astronomy. Also involved in the research were Dr. Thomas Greif of the Max Planck Institute for Astrophysics (Garching) and Prof. Dr. Volker Bromm of the University of Texas. This research was funded by the Baden-Württemberg Foundation as part of the International Top-Level Research II programme. Additional support came from the FRONTIER innovation fund of Heidelberg University as well as the German Research Foundation, the US National Science Foundation, and NASA.
Shetty, R., Glover, S. C., Dullemond, C. P., Klessen, R. S., 2011, MNRAS, 412, 1686
Modeling CO Emission: I. CO as a Column Density Tracer and the X-Factor in Molecular Clouds
Theoretical and observational investigations have indicated that the abundance of carbon monoxide (CO) is very sensitive to intrinsic properties of the gaseous medium, such as density, metallicity, and the background radiation field. CO observations are often employed to study the properties of molecular clouds (MCs), such as mass, morphology, and kinematics. It is thus important to understand how well CO traces the total mass, which in MCs is predominantly due to molecular hydrogen (H2). Recent hydrodynamic simulations by Glover & Mac Low have explicitly followed the formation and destruction of molecules in model MCs under varying conditions. These models have confirmed that CO formation strongly depends on the cloud properties. Conversely, the formation of H2 is primarily determined by the amount of time available for its formation. We apply radiative transfer calculations to these MC models in order to investigate the properties of CO line emission. We focus on integrated CO (J=1-0) intensities emerging from individual clouds, including its relationship to the total, H2, and CO column densities, as well as the ``X factor,'' the ratio of H2 column density to CO intensity. Models with high CO abundances have a threshold CO intensity ~65 K km/s at sufficiently large extinctions (or column densities). Clouds with low CO abundances show no such intensity thresholds. The distribution of total and H2 column densities are well described as log-normal functions, though the distributions of CO intensities and column densities are usually not log-normal. In general, the probability distribution functions of the integrated intensity do not follow the distribution functions of CO column densities. In the model with Milky Way-like conditions, the X factor is in agreement with the near constant value determined from observations. In clouds with lower metallicity, lower density, or a higher background UV radiation field, the CO abundances are in general lower, and hence the X factor can vary appreciably - sometimes by up to 4 orders of magnitude. In models with high densities, the CO line is fully saturated, so that the X factor is directly proportional to the molecular column density
Smith, R.J.,Glover, S.C.O.,Bonnell, I.A.,Clark, P.C., Klessen, R.S., 2011, MNRAS, 411, 1354
A quantification of the non-spherical geometry and accretion of collapsing cores
We present the first detailed classification of the structures of Class 0 cores in a high resolution simulation of a giant molecular cloud. The simulated cloud contains ten thousand solar masses and produces over 350 cores which allows for meaningful statistics. Cores are classified into three types according to how much they depart from spherical symmetry. We find that three quarters of the cores are better described as irregular filaments than as spheres. Recent Herschel results have shown that cores are formed within a network of filaments, which we find has had a significant impact on the resulting core geometries. We show that the column densities and ram pressure seen by the protostar are not uniform and generally peak along the axes of the filament. The angular momentum vector of the material in the cores varies both in magnitude and direction, which will cause the rotation vector of the central source to fluctuate during the collapse of the core. In the case of the more massive stars, accretion from the environment outside the original core volume is even more important than that from the core itself. This additional gas is primarily accreted onto the cores along the dense filaments in which the cores are embedded, and the sections of the surfaces of the cores which do not coincide with a filament have very little additional material passing through them. The assumption of spherical symmetry cannot be applied to the majority of collapsing cores, and is never a good description of how stars accrete gas from outside the original core radius. This has ramifications for our understanding of collapsing cores, in particular their line profiles, the effect of radiation upon them and their ability to fragment.
Sur, S., Schleicher, D. R. G., Banerjee, R., Federrath, C.,
Klessen, R. S.
The Astrophysical Journal Letters, Volume 721, Issue 2, pp.
L134-L138 (2010)
The Generation of Strong Magnetic Fields During the Formation of the First Stars
Cosmological hydrodynamical simulations of primordial star formation suggest that the gas within the first star-forming halos is turbulent. This has strong implications on the subsequent evolution, in particular on the generation of magnetic fields. Using high-resolution numerical simulations, we show that in the presence of turbulence, weak seed magnetic fields are exponentially amplified by the small-scale dynamo during the formation of the first stars. The two images show a snapshot of the central region of the collapsing core in our highest resolution simulation (local Jeans length resolved by 128 grid cells) at a time when the central density has increased by a factor of ∼106. Starting from a weak 1 nG field, the magnetic field grows by a factor of 106, reaching a peak value of about 1 mG at a time, τ∼ 12. The left image shows the density and the velocity structure while the right image shows the magnetic field strength and the local magnetic field directions. The circle denotes the instanteneous Jeans volume within which all dynamical quantities of interest are computed. We conclude that strong magnetic fields are generated during the birth of the first stars in the universe, potentially modifying the mass distribution of these stars and influencing the subsequent cosmic evolution. We find that the presence of the small-scale turbulent dynamo can only be identified in numerical simulations in which the turbulent motions in the central core are resolved with at least 32 grid cells.
Klessen, R. S., Hennebelle, P.: Astronomy and Astrophysics, in press (2010)
Accretion-driven turbulence as universal process: galaxies, molecular clouds, and protostellar disks
Complex turbulent motions are ubiquitously observed in many astrophysical systems. Their origin, however, is still poorly understood. When cosmic structures form, they grow in mass via accretion from the surrounding environment. We propose that this accretion is able to drive internal turbulent motions in a wide range of astrophysical objects and study this process in the case of galaxies, molecular clouds and protoplanetary disks. We use a combination of numerical simulations and analytical arguments to predict the level of turbulence as a function of the accretion rate, the dissipation scale, and the density contrast, and compare with observational data. We find that in Milky Way type galaxies the observed level of turbulence in the interstellar medium can be explained by accretion, provided that the galaxies gain mass at a rate comparable to the rate at which they form stars. This process is particularly relevant in the extended outer disks beyond the star-forming radius. We also calculate the rate at which molecular clouds grow in mass when they build up from the atomic component of the galactic gas and find that their internal turbulence is likely to be driven by accretion as well. It is the very process of cloud formation that excites turbulent motions on small scales by establishing the turbulent cascade. In the case of T Tauri disks, we show that accretion can drive subsonic turbulence at the observed level if the rate at which gas falls onto the disk is comparable to the rate at which disk material accretes onto the central star. This also explains the observed relation of accretion rate and stellar mass, dM/dt ~ M^1.8. The efficiency required to convert infall motion into turbulence is of the order of a few percent in all three cases. We conclude that accretion-driven turbulence is a universal concept with far-reaching implications for a wide range of astrophysical objects.
Federrath, C., Banerjee, R., Clark, P. C., Klessen, R. S., The Astrophysical Journal, Volume 713, Issue 1, pp. 269-290 (2010).
Modeling Collapse and Accretion in Turbulent Gas Clouds: Implementation and Comparison of Sink Particles in AMR and SPH
Star formation is such a complex process that accurate numerical tools are needed to quantitatively examine the mass distribution and accretion of fragments in collapsing, turbulent, magnetized gas clouds. To enable a numerical treatment of this regime, we implemented sink particles in the adaptive mesh refinement (AMR) hydrodynamics code FLASH. Sink particles are created in regions of local gravitational collapse, and their trajectories and accretion can be followed over many dynamical times. We perform a series of tests including the time integration of circular and elliptical orbits, the collapse of a Bonnor-Ebert sphere and a rotating, fragmenting cloud core. We compare the collapse of a highly unstable singular isothermal sphere to the theory by Shu, and show that the sink particle accretion rate is in excellent agreement with the theoretical prediction. To model eccentric orbits and close encounters of sink particles accurately, we show that a very small timestep is often required, for which we implemented subcycling of the N-body system. We emphasize that a density threshold for sink particle creation alone is insufficient for supersonic flows, if the density threshold is below the opacity limit. The density can exceed the threshold in strong shocks that do not necessarily lead to local collapse. Additional checks for bound state, gravitational potential minimum, Jeans instability and converging flows are absolutely necessary for a meaningful creation of sink particles. We apply our new sink particle module for FLASH to the formation of a stellar cluster, and compare to a smoothed particle hydrodynamics (SPH) code with sink particles. Our comparison shows encouraging agreement of gas properties, indicated by column density distributions and radial profiles, and of sink particle formation times and positions. We find excellent agreement in the number of sink particles formed, and in their accretion and mass distributions.
Simulation movies available here.
Peters, T., Banerjee, R., Klessen, R. S., Mac Low, M.-M., Galvan-Madrid, R., Keto, E. 2010, ApJ in press (arXiv:1001.2470)
H II regions: Witnesses to massive star formation
We present the first three-dimensional collapse simulations of massive star formation that include heating by both ionizing and non-ionizing radiation. Starting from a molecular cloud with 1000 Msol, we self-consistently follow its gravitational collapse, the formation of a stellar cluster and, for the first time, the dynamical evolution of the H II regions around the massive stars in the cluster. These adaptive mesh simulations have been run with the FLASH code, using a novel numerical method that couples a powerful raytracing algorithm to sink particles as a model for protostars.
The picture shows the various H II region morphologies that can be observed within the cluster. We find that the H II regions are highly variable in time and shape, taking on any morphological form known from surveys (shell-like, core-halo, cometary, spherical, irregular) in a single simulation. In particular, we find that the observed morphology depends solely on the structure of the flow field and the viewing angle onto the H II region. The shown images represent synthetic VLA observations of the simulation data at a wavelength of 2 cm. The black dots are stars, represented by sink particles.
Glover, S. C. O., Federrath, C., Mac Low, M.-M., Klessen, R. S. 2009, Monthly Notices of the Royal Astronomical Society, in press.
Modelling CO formation in the turbulent interstellar medium
We present results from high-resolution three-dimensional simulations of turbulent interstellar gas that self-consistently follow its coupled thermal, chemical and dynamical evolution, with a particular focus on the formation and destruction of H2 and CO. We quantify the formation timescales for H2 and CO in physical conditions corresponding to those found in nearby giant molecular clouds, and show that both species form rapidly, with chemical timescales that are comparable to the dynamical timescale of the gas.
The picture shows: (a) Column density of hydrogen nuclei, NH,tot, in run R3 at time t = tend, viewed along a line of sight parallel to the z-axis of the simulation volume. This direction is also parallel to the initial orientation of the magnetic field. (b) As (a), but for the H2 column density. (c) As (a), but for the CO column density. (d) Ratio of H2 column density to CO column density along the same line of sight through run R3 at time t = tend. The last plot in particular shows that CO does clearly not trace the gas density in all regions in a 1:1 fashion.
The gas has a filamentary distribution, and large spatial variations in the column densities are apparent, including, coincidentally, a rather prominent under-density visible toward the bottom-left of the figures. The plots of NH,tot and NH2 are very difficult to distinguish, which is unsurprising since most of the hydrogen gas in the simulation is molecular by this point. On the other hand, the plot of CO column density is clearly different from the other two plots: the underdense regions are larger, and also more numerous, particularly towards the edges of the box.
Jappsen, A.-K., Klessen, R. S., Glover, S. C. O., Mac Low, M.-M. 2009, Astrophysical Journal, Volume 696, Issue 2, pp. 1065-1074.
Star Formation at Very Low Metallicity. IV. Fragmentation does not Depend on Metallicity for Cold Initial Conditions
Primordial star formation appears to result in stars at least an order of magnitude more massive than modern star formation. It has been proposed that the transition from primordial to modern initial mass functions occurs due to the onset of effective metal-line cooling at a metallicity Z/Zsun = 10-3.5. However, these simulations neglected molecular hydrogen cooling. We perform simulations using the same initial conditions, but including molecular cooling, using a complex network that follows molecular hydrogen formation and also directly follows carbon monoxide and water. The image shows a projection of one of our simulated protogalaxies at a redshift of 35.
Our simulations show that molecular hydrogen cooling allows roughly equivalent fragmentation to proceed even at zero metallicity for these initial conditions. The apparent transition seen in previous studies simply represents the point where metal-line cooling becomes more important than molecular cooling. In all of the cases that we have studied, the fragments are massive enough to be consistent with models of primordial stellar masses, suggesting that the transition to the modern initial mass function may be determined by other physics such as dust formation. We conclude that such additional cooling mechanisms, combined with the exact initial conditions produced by cosmological collapse are likely more important than metal-line cooling in determining the initial mass function, and thus that there is unlikely to be a sharp transition in the initial mass function at Z/Zsun = 10-3.5.
Dobbs, C. L., Glover, S. C. O., Clark, P. C., Klessen, R. S. 2009, Monthly Notices of the Royal Astronomical Society, Volume 389, Issue 3, pp. 1097 - 1110.
The ISM in Spiral Galaxies: Can Cooling in Spiral Shocks Produce Molecular Clouds?
We investigate the thermodynamics of the interstellar medium (ISM) and the formation of molecular hydrogen through numerical simulations of spiral galaxies. The model follows the chemical, thermal and dynamical response of the disc to an external spiral potential. Self-gravity and magnetic fields are not included. The calculations demonstrate that gas can cool rapidly when subject to a spiral shock. Molecular clouds in the spiral arms arise through a combination of compression of the ISM by the spiral shock and orbit crowding. These results highlight that local self-gravity is not required to form molecular clouds. Self-shielding provides a sharp transition density, below which gas is essentially atomic, and above which the molecular gas fraction is >0.001. The time-scale for gas to move between these regimes is very rapid (<=1 Myr). From this stage, the majority of gas generally takes between 10 and 20 Myr to obtain high-H2 fractions (>50 per cent). These are, however, strict upper limits to the H2 formation time-scale, since our calculations are unable to resolve turbulent motions on scales smaller than the spiral arm, and do not include self-gravity. True cloud formation time-scales are therefore expected to be even shorter.
The mass budget of the disc is dominated by cold gas residing in the spiral arms. Between 50 and 75 per cent of this gas is in the atomic phase. When this gas leaves the spiral arm and drops below the self-shielding limit, it is heated by the galactic radiation field. Consequently, most of the volume in the interarm regions is filled with warm atomic gas. However, some cold spurs and clumps can survive in interarm regions for periods comparable to the interarm passage time-scale. Altogether between 7 and 40 per cent of the gas in our disc is molecular, depending on the surface density of the calculation, with approximately 20 per cent molecular for a surface density comparable to the solar neighbourhood.
Schleicher Dominik R. G., Glover Simon C. O., Banerjee Robi, Klessen Ralf S. 2009, PRD, 79, 023515
Cosmic constraints rule out S-wave annihilation of light dark matter
Light dark matter is discussed as one possible explanation for the observed 511 keV line emission in the Galactic center. This emission correlates with the Galactic bulge rather than the disk and is difficult to explain with astrophysical sources. The light dark matter model assumes that dark matter particles of a few MeV annihilate into electron-positron pairs, which can then annihilate further and produce 511 keV photons. The annihilation process is accompanied by the release of internal bremsstrahlung. It was shown previously that this model may even overpredict the observed flux if a velocity-independent cross section is adopted.
We have examined the model in the cosmological context, calculating the expected gamma-ray backgrounds due to 511 keV line emission as well as the internal bremsstrahlung emission. This allows to constrain the clumpiness of dark matter, as the annihilation rate is proportional to the dark matter density squared, and is therefore enhanced if dark matter clusters in steep clumps. These constraints are shown as a function of mass in the above figure. The annihilation cross section is fixed by requiring that the observed dark matter abundance forms in thermal equilibrium in the early universe. The quantity C0 is the clumping factor of dark matter at redshift zero, and is given as the average of the squared dark matter density divided by the mean dark matter density squared. The grey-shaded region is excluded due to this constraint. In addition, we plot the minimal clumping factor expected in current models for dark matter, as well as the upper limit on the light dark matter mass derived from the spectral shape of the flux emitted in the galactic center. The combination of these constraints rules out the light dark matter model for a velocity-independent cross section.
Bonnell Ian A., Clark Paul C., Bate Matthew R. 2008, MNRAS, 389, 1556 (arXiv:0807.0460)
Gravitational fragmentation and the formation of brown dwarfs in stellar clusters
We investigate the formation of brown dwarfs and very low mass stars through the gravitational fragmentation of infalling gas into stellar clusters. The gravitational potential of a forming stellar cluster provides the focus that attracts gas from the surrounding molecular cloud. Structures present in the gas grow, forming filaments flowing into the cluster centre. These filaments attain high gas densities due to the combination of the cluster potential and local self-gravity. The resultant Jeans masses are low, allowing the formation of very low mass fragments. The tidal shear and high-velocity dispersion present in the cluster preclude any subsequent accretion, thus resulting in the formation of brown dwarfs or very low mass stars. Ejections are not required as the brown dwarfs enter the cluster with high relative velocities, suggesting that their disc and binary properties should be similar to that of low-mass stars. This mechanism requires the presence of a strong gravitational potential due to the stellar cluster implying that brown-dwarf formation should be more frequent in stellar clusters than in distributed populations of young stars. Brown dwarfs formed in isolation would require another formation mechanism such as due to turbulent fragmentation.
The image shows a position-velocity diagram for a gaseous filament falling into a cluster, centred on the site of clump of gas that is about to collapse to become a brown dwarf. The velocities are calculated projected along the vector joining the brown dwarf at position x = 0 to the centre of mass of the cluster, and are again centred on the brown dwarf. The diagonal form of the filament in position-velocity diagram reveals that the gas is accelerating into the cluster while being tidally sheared away from the objects that are forming within. Relative to the formation site of the brown dwarf, the vast majority of the filament is moving away: gas at negative positions has negative relative velocities, while gas at positive positions has positive relative velocities. Only in the immediate region surrounding the still-forming brown dwarf can one see a reversal of this velocity signature, which denotes the gas falling on to the new object. This feature can be seen in several other points along the filament, showing the formation sites of other brown dwarfs or low-mass stars. Relative to the formation site of the brown dwarf, the vast majority of the filament is moving away: gas at negative positions has negative relative velocities, while gas at positive positions has positive relative velocities. Only in the immediate region surrounding the still-forming brown dwarf can one see a reversal of this velocity signature, which denotes the gas falling on to the new object. This feature can be seen in several other points along the filament, showing the formation sites of other brown dwarfs or low-mass stars.
Banerjee R., Vazquez-Semadeni E., Hennebelle P., Klessen R. S., MNRAS, submitted (arXiv:0808.0986)
The early stages of molecular cloud evolution in the magnetised interstellar medium: clump morphology and evolution
We study the properties of clumps formed in three-dimensional simulations of converging flows in the magnetised, thermally bistable, warm neutral medium (WNM). Based on these simulations we find: (1) Pockets of cold, dense molecular clumps form through thermal instability in the contact layer of the convergent flow. They are characterised by a sharp density jump at the boundary to the WNM. (2) The clumps grow in mass at roughly constant density by the outward propagation of the sharp transition layer to the WNM and, at later times, by coagulation. (3) The clumps are approximately in pressure equilibrium with their surroundings and are well separated in the early stages of the cloud evolution. They typically have mean densities of n ~ 1000 cm^-3 and temperatures of T ~ 23 K. (4) The clumps internal turbulence is transonic with pressure fluctuations of up to factors of 2 above and below the thermal value of the surrounding WNM. (5) The velocity and magnetic fields tend to be aligned with each other within the clumps, although both are significantly fluctuating, suggesting that the velocity tends to stretch and align the magnetic field with it. We conclude that thermal instability is responsible for the large density contrast between clumps and WNM, while turbulent ram pressure fluctuations together with gravity are responsible for the internal substructure of the clumps. In the final stages of the evolution mergers drive clumps into the gravitationally unstable regime and consequently star formation sets in.
The picture shows the face-on view of column density from the weakly magnetized colliding flow simulations. High density clumps of cold gas form through thermal instabilities out of the warm neutral medium. Eventual these clumps become gravitationally unstable and start to collapse (indicated by the black dots).
Simulation movies available here.
Federrath C., Klessen R. S., Schmidt W., 2008, ApJL, 688, L79 (arXiv:0808.0605)
The Density Probability Distribution in Compressible Isothermal Turbulence: Solenoidal vs Compressive Forcing
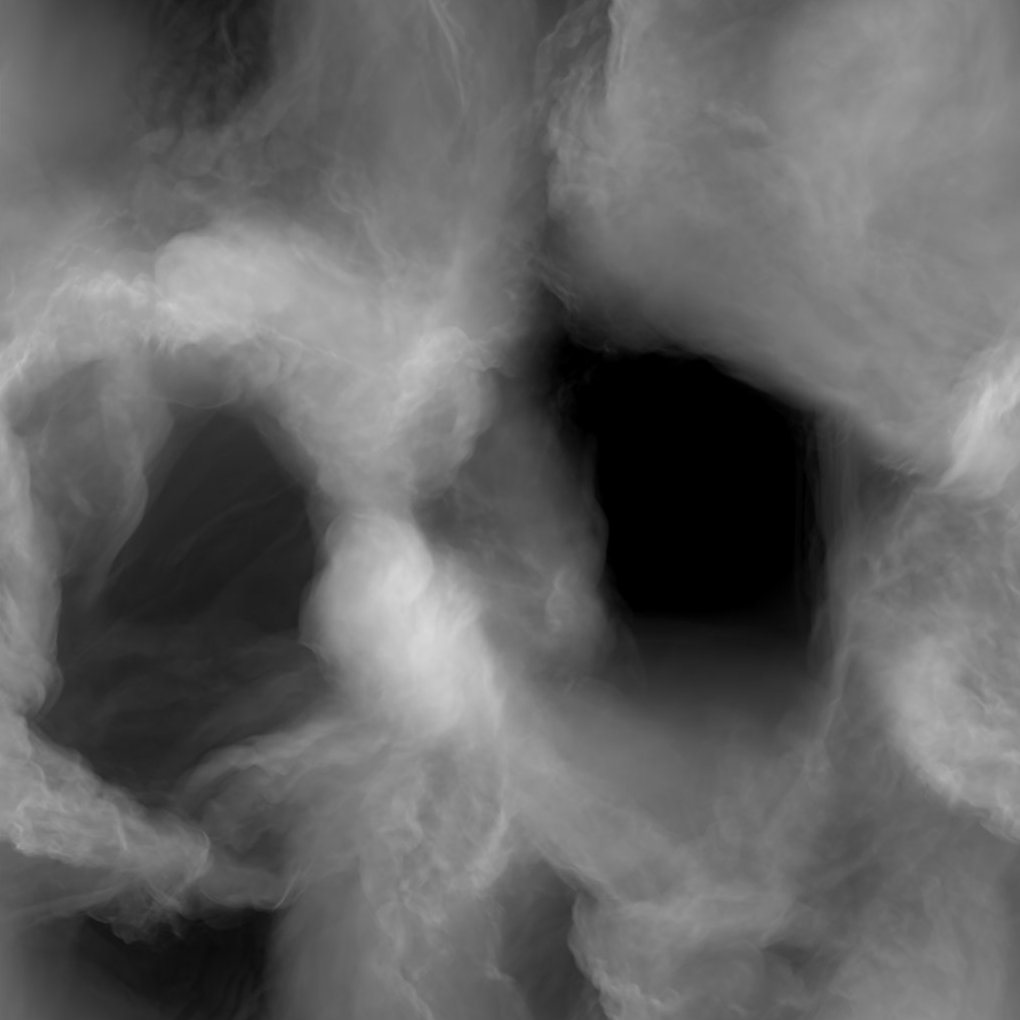
The probability density function (PDF) of the gas density in turbulent supersonic flows is investigated with high-resolution numerical simulations. In a systematic study, we compare the density statistics of compressible turbulence driven by the usually adopted solenoidal forcing (divergence-free) and by compressive forcing (curl-free). Our results are in agreement with studies using solenoidal forcing. However, compressive forcing yields a significantly broader density distribution with standard deviation ~3 times larger at the same rms Mach number. The standard deviation-Mach number relation used in analytical models of star formation is reviewed and a modification of the existing expression is proposed, which takes into account the ratio of solenoidal and compressive modes of the turbulence forcing.
The picture on the right provides a column density map of the turbulence simulation using compressive forcing at rms Mach 5. Clicking on the image brings up a comparison of the same type of map from a simulation using solenoidal forcing. Obviously, the density statistics differ in both cases although the rms Mach number was kept constant. This shows that the density statistics not only depends on the rms Mach number, but also on the turbulence forcing.
Simulation movies available here.
Schmeja S., Kumar M.S.N., Ferreira B., 2008, MNRAS, 389, 1209
The structure of a nascent star cluster
The young stellar population data of the Perseus, Ophiuchus and
Serpens molecular clouds are obtained from the Spitzer c2d legacy survey
in order to investigate the spatial structure of embedded clusters
using the nearest neighbour and minimum spanning tree method. We
identify the embedded clusters in these clouds as density
enhancements and analyse the clustering parameter Q with
respect to source luminosity and evolutionary stage. This analysis
shows that the older Class 2/3 objects are more centrally
condensed than the younger Class 0/1 protostars, indicating
that clusters evolve from an initial hierarchical configuration to
a centrally condensed one. Only IC 348 and the Serpens core,
the older clusters in the sample, shows signs of mass segregation
(indicated by the dependence of Q on the source
magnitude), pointing to a significant effect of dynamical
interactions after a few Myr. The structure of a cluster may also
be linked to the turbulent energy in the natal cloud as the most
centrally condensed cluster is found in the cloud with the lowest
Mach number and vice versa. In general these results agree well
with theoretical scenarios of star cluster formation by
gravoturbulent fragmentation.
The picture shows the 20th nearest neighbour density of the young
stars in L1688, the main cluster in the Ophiuchus molecular cloud.
This is an example of a highly hierarchically structured cluster
showing several density peaks and at least three readily
identifiable subclusters. The thick line indicates the cluster
boundary (defined as 3 sigma above the background density),
the star symbols show the positions of two massive B stars.
(Click on the image for a larger view.)
Download preprint
(also available at arXiv:0805.2049)
Published article: MNRAS 389,
1209 (2008)
Greif T. H., Johnson J. L., Klessen R. S., Bromm V. 2008, MNRAS, 387, 1021 (arXiv:0803.2237)
The First Galaxy
We investigate the properties of the first galaxies at z > 10
with highly resolved numerical simulations, starting from
cosmological initial conditions and taking into account all
relevant primordial chemistry and cooling. A first galaxy is
characterized by the onset of atomic hydrogen cooling, once the
virial temperature exceeds 10^4 K, and its ability to retain
photoheated gas. We follow the complex accretion and star
formation history of a 5*10^7 M_sun system by means of a detailed
merger tree and derive an upper limit on the number of Population
III stars formed prior to its assembly. We investigate the thermal
and chemical evolution of infalling gas and find that partial
ionization at temperatures > 10^4 K catalyzes the formation of
H2 and HD, allowing the gas to cool to the temperature of the CMB.
Depending on the strength of radiative and chemical feedback,
primordial star formation might be dominated by intermediate-mass
Pop III stars formed during the assembly of the first galaxies.
Accretion onto the nascent galaxy begins with hot accretion, where
gas is accreted directly from the IGM and shock-heated to the
virial temperature, but is quickly accompanied by a phase of cold
accretion, where the gas cools in filaments before flowing into
the parent halo with high velocities. The latter drives supersonic
turbulence at the center of the galaxy and could lead to very
efficient chemical mixing. The onset of turbulence in the first
galaxies thus likely marks the transition to Pop II star
formation.
More material can be found here.
Clark, Glover and Klessen, 2008, ApJ, 672, 757
The First Stellar Cluster
We report
results from numerical simulations of star formation in the early
universe that focus on gas at very high densities and very low
metallicities. We argue that the gas in the central regions of
protogalactic halos will fragment as long as it carries sufficient
angular momentum. Rotation leads to the build-up of massive
disk-like structures which fragment to form protostars. At
metallicities Z ~ 10^-5 Zsun, dust cooling becomes effective and
leads to a sudden drop of temperature at densities above n = 10^12
cm^-3. This induces vigorous fragmentation, leading to a very
densely-packed cluster of low-mass stars. This is the first
stellar cluster. The mass function of stars peaks below 1 Msun,
similar to what is found in the solar neighborhood, and comparable
to the masses of the very-low metallicity subgiant stars recently
discovered in the halo of our Milky Way. We find that even purely
primordial gas can fragment at densities 10^14 cm^-3 < n <
10^16 cm^-3, although the resulting mass function contains only a
few objects (at least a factor of ten less than the Z = 10^-5 Zsun
mass function), and is biased towards higher masses. A similar
result is found for gas with Z = 10^-6 Zsun. Gas with Z <=
10^-6 Zsun behaves roughly isothermally at these densities (with
polytropic exponent gamma ~ 1.06) and the massive disk-like
structures that form due to angular mom entum conservation will be
marginally unstable. As fragmentation is less efficient, we expect
stars with Z <= 10^-6 Zsun to be massive, with masses in excess
of several tens of solar masses, consistent with the results from
previous studies
This work has now been published, and the offical version can be found here . Please feel free to contact the authors for more information or off-prints.